New software will allow creation of more complex devices.
Someday, scientists believe, tiny DNA-based robots and other nanodevices will deliver medicine inside our bodies, detect the presence of deadly pathogens, and help manufacture increasingly smaller electronics.
Researchers took a big step toward that future by developing a new tool that can design much more complex DNA robots and nanodevices than were ever possible before in a fraction of the time.
In a paper published on April 19, 2021, in the journal Nature Materials, researchers from The Ohio State University – led by former engineering doctoral student Chao-Min Huang – unveiled new software they call MagicDNA.
The software helps researchers design ways to take tiny strands of DNA and combine them into complex structures with parts like rotors and hinges that can move and complete a variety of tasks, including drug delivery.
Researchers have been doing this for a number of years with slower tools with tedious manual steps, said Carlos Castro, co-author of the study and associate professor of mechanical and aerospace engineering at Ohio State.
“But now, nanodevices that may have taken us several days to design before now take us just a few minutes,” Castro said.
And now researchers can make much more complex – and useful – nanodevices.
“Previously, we could build devices with up to about six individual components and connect them with joints and hinges and try to make them execute complex motions,” said study co-author Hai-Jun Su, professor of mechanical and aerospace engineering at Ohio State.
“With this software, it is not hard to make robots or other devices with upwards of 20 components that are much easier to control. It is a huge step in our ability to design nanodevices that can perform the complex actions that we want them to do.”
A robot arm nanodevice with claw that can pick up smaller items. Credit: Ohio State University
The software has a variety of advantages that will help scientists design better, more helpful nanodevices and – researchers hope – shorten the time before they are in everyday use.
One advantage is that it allows researchers to carry out the entire design truly in 3D. Earlier design tools only allowed creation in 2D, forcing researchers to map their creations into 3D. That meant designers couldn’t make their devices too complex.
The software also allows designers to build DNA structures “bottom up” or “top down.”
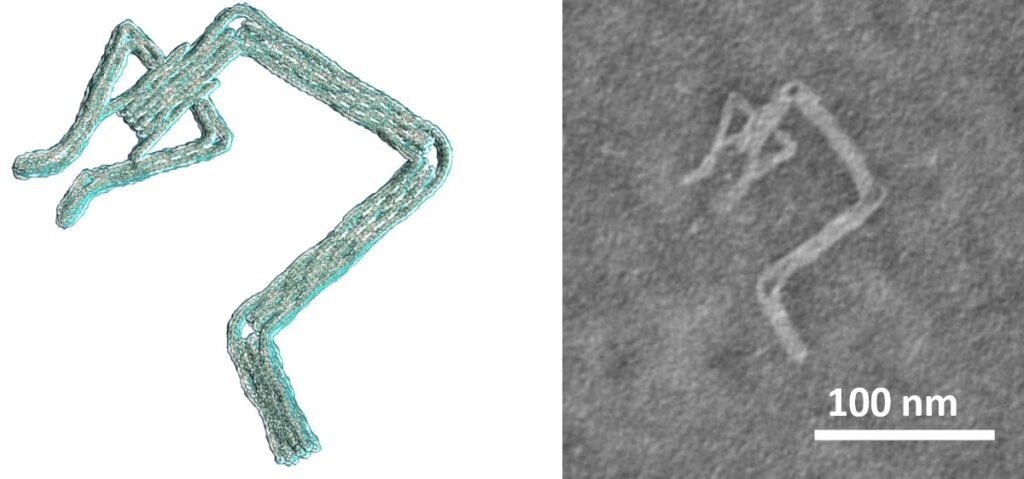
In “bottom up” design, researchers take individual strands of DNA and decide how to organize them into the structure they want, which allows fine control over local device structure and properties.
But they can also take a “top down” approach where they decide how their overall device needs to be shaped geometrically and then automate how the DNA strands are put together.
Combining the two allows for increasing complexity of the overall geometry while maintaining precise control over individual component properties, Castro said.
Another key element of the software is that it allows simulations of how designed DNA devices would move and operate in the real world.
“As you make these structures more complex, it is difficult to predict exactly what they are going to look like and how they are going to behave,” Castro said.
“It is critical to be able to simulate how our devices will actually operate. Otherwise, we waste a lot of time.”
As a demonstration of the software’s ability, co-author Anjelica Kucinic, a doctoral student in chemical and biomolecular engineering at Ohio State, led the researchers in making and characterizing many nanostructures designed by the software.
Some of the devices they created included robot arms with claws that can pick up smaller items, and a hundred nanometer-sized structure that looks like an airplane (The “airplane” is 1000 times smaller than the width of a human hair).
The ability to make more complex nanodevices means that they can do more useful things and even carry out multiple tasks with one device, Castro said.
For example, it is one thing to have a DNA robot that, after injection into the bloodstream, can detect a certain pathogen.
“But a more complex device may not only detect that something bad is happening, but can also react by releasing a drug or capturing the pathogen,” he said.
“We want to be able to design robots that respond in a particular way to a stimulus or move in a certain way.”
Castro said he expects that for the next few years, the MagicDNA software will be used at universities and other research labs. But its use could expand in the future.
“There is getting to be more and more commercial interest in DNA nanotechnology,” he said. “I think in the next five to 10 years we will start seeing commercial applications of DNA nanodevices and we are optimistic that this software can help drive that.”
Reference: “Integrated computer-aided engineering and design for DNA assemblies” by Chao-Min Huang, Anjelica Kucinic, Joshua A. Johnson, Hai-Jun Su and Carlos E. Castro, 19 April 2021, Nature Materials.
Joshua Johnson, who received his PhD at Ohio State in biophysics, was also a co-author of the paper.
The research was supported by grants from the National Science Foundation.